Overview
The ushering in of the new digital era (Fourth Industrial Revolution) and a hyper-connected society has accelerated in recent times through necessity as our society rapidly embraces digitalization for remote working, education, telehealth, video-conferencing and many other aspects of our daily lives.
At the same time adoption of new technologies, including 5G, IOT/ IIOT, AI, autonomous vehicles and edge-computing, is defining a whole new paradigm for how, where and why data and information is consumed, distributed and stored. The steadfast demand impels an ever-increasing drive to push the performance and capabilities of new and existing technologies even further.
In the semiconductor industry, challenges in the scalability of Moore’s law are driving the industry to develop 2.5D/3D-IC and advanced packaging technologies for performance improvements. Micro-Electro-Mechanical Systems (MEMS) devices are currently powering countless sensors in smartphones, microphones of smart speakers/devices and in the near future, the possibility of electronic noses as well. Silicon photonics provide the bandwidth required to handle the data-hungry demands of 5G, AI and IOT/IIOT in hyperscale data centers. Advanced fluorescence in the second Near-Infrared (NIR-II) region allows improvements in deep tissue penetration in-vivo optical imaging otherwise limited to optical scattering.
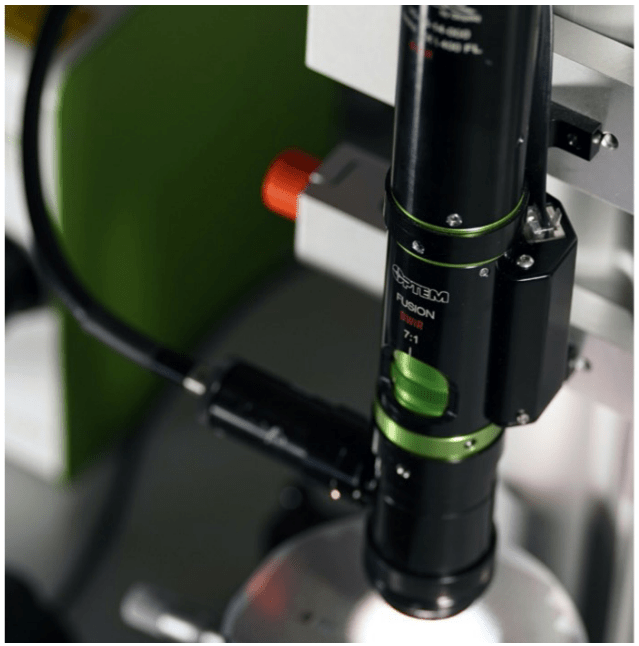
All these technologies benefit from the extended capabilities of Short-Wave Infrared (SWIR) microscopy. This article explores these applications in further detail and how SWIR microscopes, like the Optem FUSION modular microscope (see Figure 1), offer a flexible advantage in SWIR imaging.
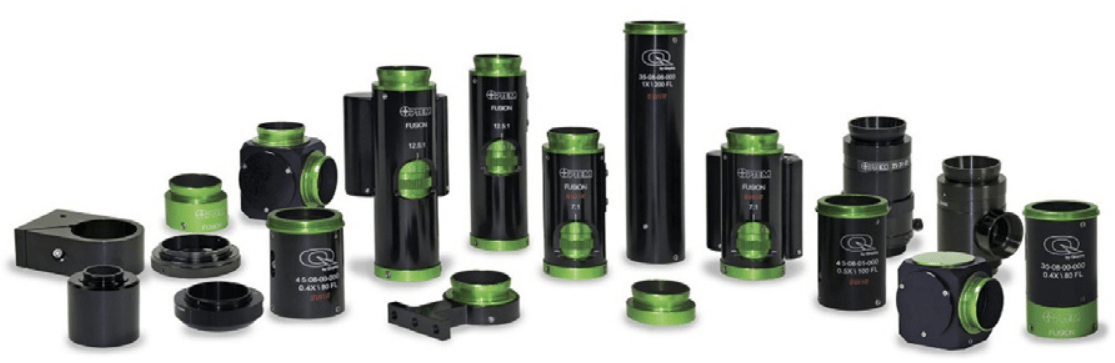
SWIR Microscopy
Classic microscopes operate in the visible wavelength range of 400-700 nm and often contain a set of viewing oculars and a camera port. With the proliferation of smartphone cameras, demand for economical high-quality images in a wide range of conditions and under extreme size, weight and power constraints has drastically improved Complementary Metal-Oxide-Semiconducter (CMOS) sensor performance. Subsequently, high-performance sensors suitable for microscopy applications also benefited and the move towards digital microscopy systems gained further momentum.
With a wide range of readily available optical modules, Excelitas’ Optem FUSION modular system provides a flexible digital microscopy platform to meet the requirements of many micro-imaging applications. The versatile system supports visible, Near Infrared (NIR), and SWIR wavelength ranges and can be configured with fixed magnification or zoom (7:1 or 12.5:1). The system also provides numerous motorized modules to meet the requirements of high throughput and automated applications.
Further advancements in alternative detector materials, manufacturing technologies and application needs opened up the possibility to image at wavelengths outside of the classic visible window. Xenics Infrared Solutions (www. xenics.com), a leading provider of infrared imaging sensors, recently released its Wildcat 640, a compact SWIR Camera (55 x 55 x 91.5 mm3) available with CameraLinkTM or USB3 interface, based on an InGaAs platform to achieve high-performance imaging in the shortwave infrared wavelength range (900 nm – 1700 nm). Able to run up to 220 Hz and offering two gains so that the users benefits from low noise
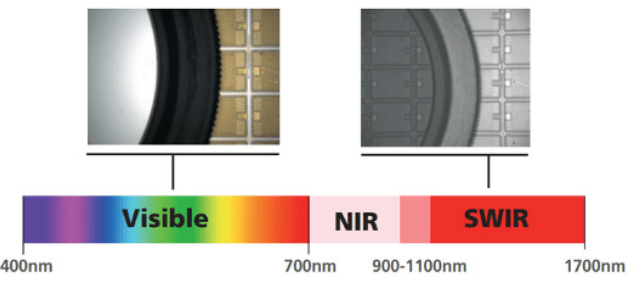
Figure 3: Extending imaging into the SWIR wavebands provides additional information not available in the visible waveband
(only 80 electrons in high gain) with a high dynamic range (63 dB in high gain mode and 68 dB in High Dynamic Range mode), Wildcat 640 offers a very flexible solution for SWIR microscopy.
Combining a SWIR Wildcat 640 Camera with an Optem FUSION modular microscope enables an entirely new range of applications and technologies not otherwise possible (Figure 3).
Applications
Semiconductor Inspection
Chip-scaling and advancing nodes to increase transistor density and computing performance face increasingly difficult physical challenges. Emphasis is shifting to other areas to achieve lower power consumption and increased speed including 2.5D/3D-ICs and advanced packaging techniques. Many of these rely on complex stacking and bonding of multiple dies or wafers with vertical interconnects (Figure 4, Figure 5).
Silicon, the backbone to the majority of electronic devices, is opaque at visible wavelengths, limiting inspection of the stacked and bonded layers to surface structures. However, at wavelengths larger than the bandgap of silicon (1100 nm), the silicon is transparent. Utilizing an Optem FUSION SWIR microscope affords the ability to see into the layers for alignment, quality assessment and defect inspection. Additionally, SWIR microscopes enable precision alignment during pre-dicing operations, followed by die defect and crack inspection of dies post-dicing.
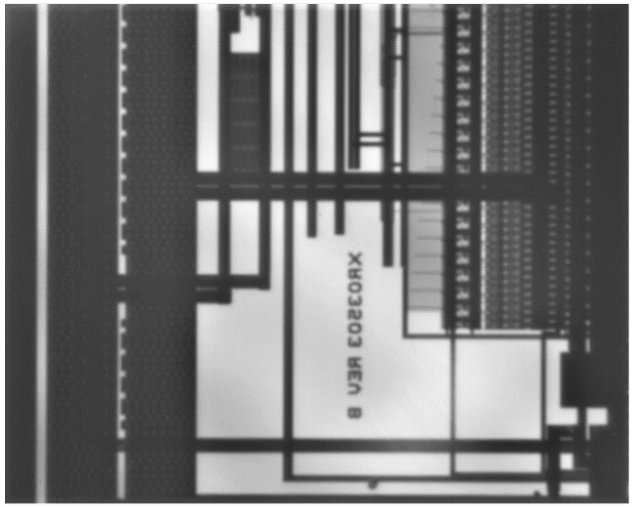
Figure 4: SWIR imaging through semiconductor chip highlighting buried features not seen with visible wavelengths
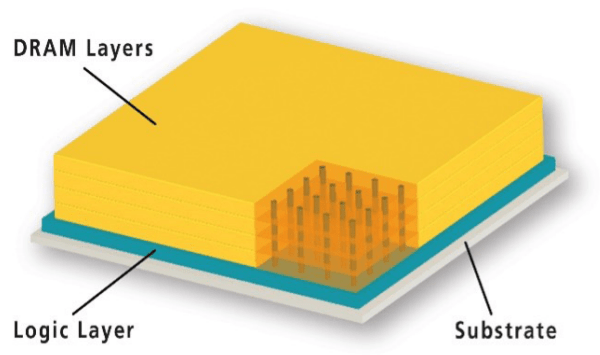
Figure 5: High Bandwidth Memory employing 3D Stacking with Interconnected Layers
MEMS
The high sensitivity, reliability and cost effectiveness of MEMS sensors are powering many features in smartphones, automobiles, advanced displays and medical devices. RF MEMS for 5G and sub-6 GHz band rollout and MEMs mics are driving demand for these micro-structured devices.
MEMS device manufacturing relies on selective patterning, etching and releasing of silicon layers, often forming membranes or cantilevers. Inspection of engineered structures and inspection after bonding are critical in manufacturing for detection of bubbles and breaches.
Today industrial printheads (for industrial printing and 3D manufacturing) utilize MEMS membranes. Inspection of the printhead for dirt/debris/bubbles is critical to quality control. SWIR Microscopy enables inspection of bubbles, voids and defects through the silicon for improved quality control (Figure 6).
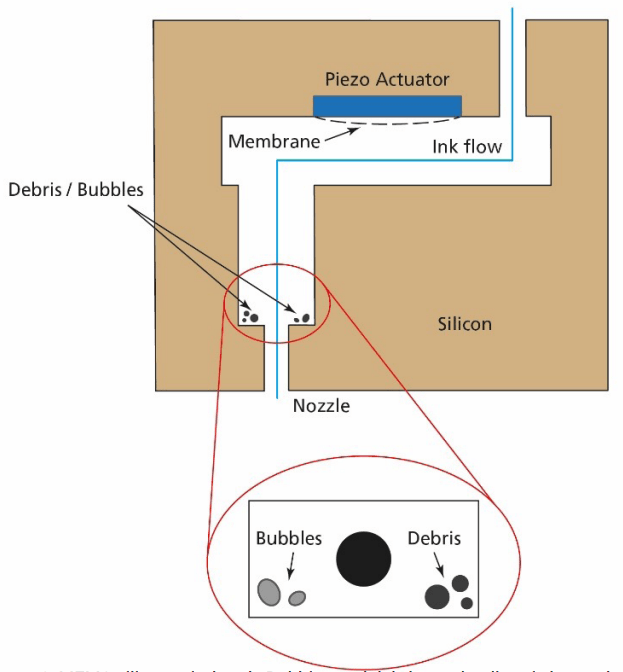
Figure 6: MEMS silicon printhead. Bubbles and debris can be directly imaged through silicon with SWIR microscopy
Silicon Photonics
Our appetite for data is growing exponentially as device interconnectivity is rapidly adopted due to societal market pulls and technological pushes including 5G and IOT/IIOT. The extreme amount of data requires immense datacenters with incredible bandwidth, pushing traditional electrical connections to their limits.
Integration of optical components onto silicon wafers using VSLI techniques enables high bandwidth (100 Gb/s today and 400 Gb/s and beyond to come) silicon photonic transceivers, key to the bandwidth demands of datacenters.
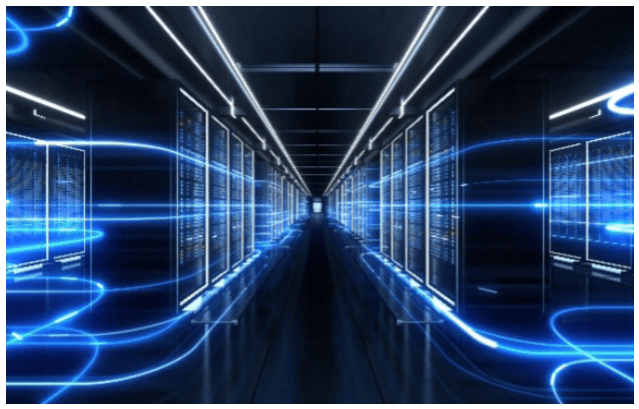
Silicon photonic devices propagate optical signals at SWIR wavelengths via integrated silicon (or Si) waveguides. Since lasers cannot be produced in silicon (Si is not a direct bandgap material), separate direct bandgap III-V laser dies (such as InP or GaN) need to be accurately flip-chip assembled and aligned onto the silicon photonic chip. SWIR microscopes provide the means to enable high-precision manufacturing, alignment, packaging and testing of these photonic integrated circuits.
Biomedical Fluorescence
Understanding the interactions and functions of living and fixed cells within the human body is critical for medical advancement. Fluorescence microscopy enables selective imaging of proteins and other biological molecules, providing revolutionary opportunities for DNA sequencing, understanding live cell interactions and drug delivery, to name only a few innovations. Tremendous efforts to improve optical sectioning and provide deeper tissue penetration, including confocal and light sheet microscopy, have been developed but still face some fundamental challenges due to absorption and scattering of visible wavelengths. Utilizing SWIR wavelengths (commonly defined as the NIR-I
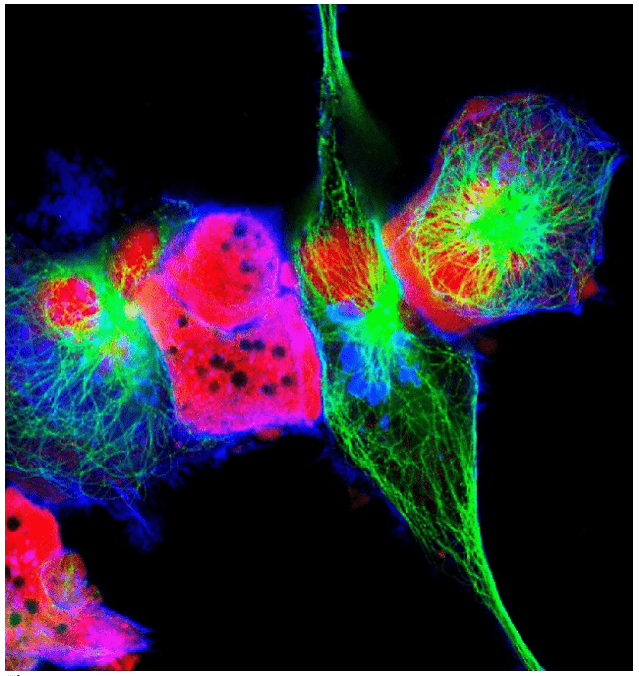
and NIR-II bands in medical applications) has many advantages including reduced absorption by blood and tissue, less scattering and a general lack of autofluorescence. This enables deeper penetration into tissue, improved image clarity and witnessing of interactions that were previously unachievable. Recent progress in quantum dot, carbon nanotubes and other fluorescent dyes are creating new opportunities for SWIR fluorescent microscopy adoption.
Additional Application Areas for SWIR Microscopy
In addition to the applications addressed above, SWIR microscopy opens up new possibilities in many other application areas including:
Materials Research
Material research for next-generation devices including carbon nanotubes, quantum dots, graphene, and borophene, exhibit extremely interesting properties that benefit from SWIR microscopy analysis.
Counterfeiting and Forensics
Inspecting artwork, currency and products in the SWIR spectral range provides additional information and subsurface inspection for authentication of counterfeits.
Microfluidics
The manufacturing and testing of microfluidic devices with SWIR wavebands enables detection of moisture absorption properties of liquids (e.g., water).
Hyperspectral Imaging
Many materials have unique spectral fingerprints in the SWIR wavelength ranges (i.e. plastics). Hyperspectral imaging with InGaAs sensors provides additional means of characterizing materials not feasible in the visible spectrum alone.